Teaming Up to Understand the Brain
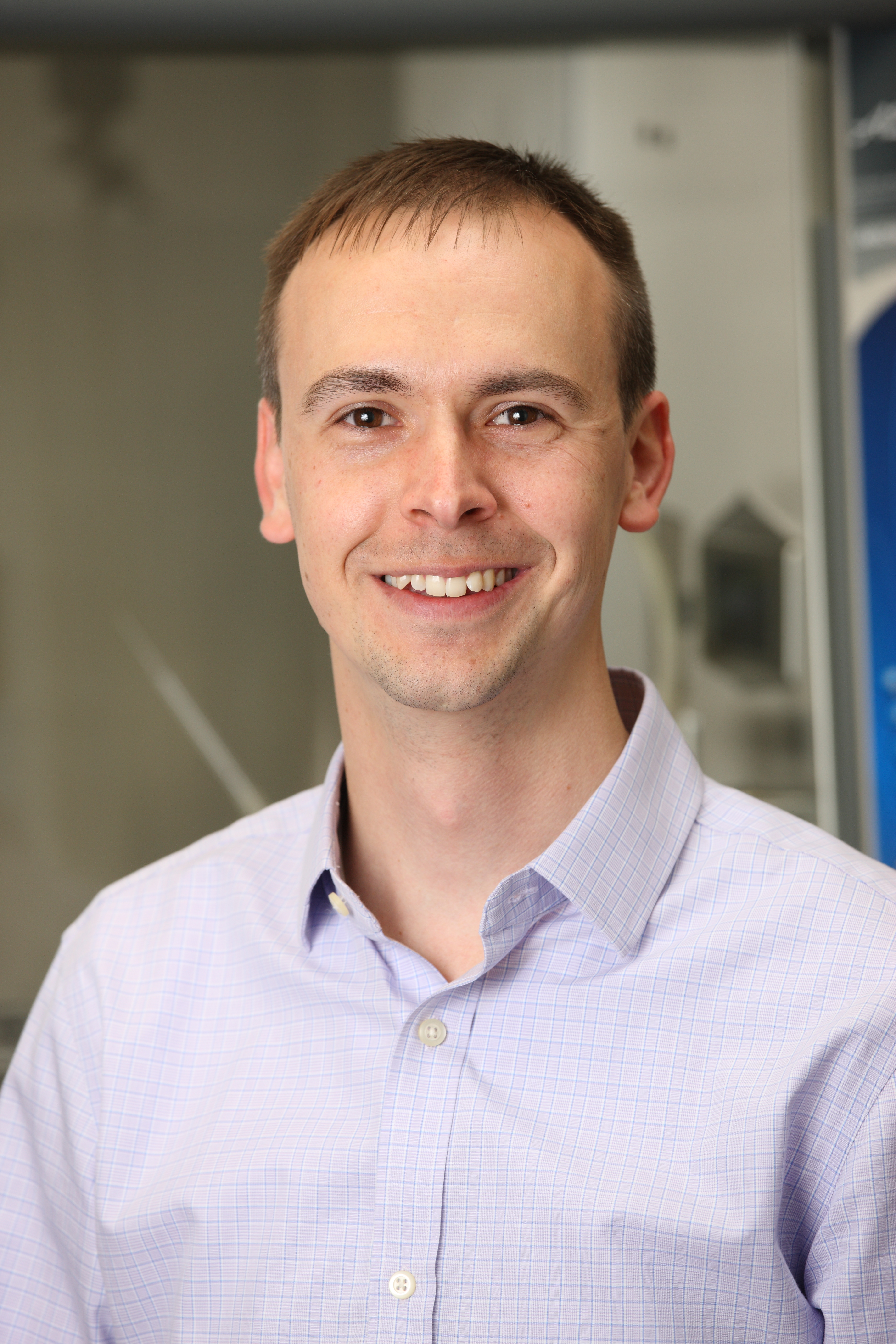
Kyle Lampe is an Associate Professor in the Department of Chemical Engineering at the University of Virginia, and by courtesy, Biomedical Engineering and Neuroscience. The Lampe Group investigates biomaterials for tissue engineering, regenerative medicine, and drug delivery within the central nervous system. Having grown up in rural Iowa, Professor Lampe is a proud supporter of engineers from first-generation, low-income, and rural families. He also takes great pleasure in mentoring undergraduate and graduate students in his lab, particularly female engineers, which constitute over half of his lab.
I would argue that research is THE most unadulterated exploratory (and for me, joyous) career path. I get to lead, mentor, and support a team of 15-20 undergraduate and graduate student researchers in our quest to engineer and understand brain tissue and address diseases and injuries like ischemic stroke, traumatic brain injury, and multiple sclerosis. Sure, we fail (a lot) in our research objectives – the brain is complicated! However, you only really fail if you don’t learn something in the process…and we are learning soooo much about brain cells, even if it’s not what we initially expected. This may surprise you, but my “professor” job is as much about learning as it is about teaching!
Most people are familiar with at least one brain cell type: neurons. Neurons have been celebrated as the “functional” cells in the brain since Ramon y Cajal observed them in the early 20th century. Largely ignored until this century are the numerous other brain cell types, like glia. Glia is Latin for “glue,” named for their original perception as the stuff that holds neurons together. UVA has a host of experts researching these formerly underappreciated glia; one of many factors that led me to UVA. Additional brain cell types include stem and precursor/progenitor cells that are critical to brain repair and regeneration. The Lampe Biomaterials group likes to think mostly about those stem and progenitor cells that can become a specific type of glia: regenerating oligodendrocytes. Oligodendrocytes make the myelin insulation in the brain, necessary for proper signal transmission, and are the cells that go haywire in conditions like multiple sclerosis.
You may be asking yourself, “Why is a chemical engineer talking about neuroscience?” My response: “Why not?”
Chemical engineers have a unique picture of how the world works because we are trained in molecular-level techniques, quantitative methods, and system dynamics to understand the movement of matter and energy, how chemical reactions work, and what drives all of this to happen. In my case, the materials and energy just happen to be specific to biological systems.
Our strategy of building cell niches from the molecule up merges with the understanding gained from collaborators who study glia in complicated whole organisms. Clearly, we don’t do this alone – our group includes students from chemical engineering, biomedical engineering, chemistry, biology, and neuroscience. And we collaborate with medical doctors in vascular neurology and anesthesiology. We have the most to learn from those we have the least in common with, which I find to be true both scientifically and personally.
I tell students who join our research group that they will 1. Make something and 2. Grow something. This epitomizes my philosophy that, while we create new biomaterial systems, we focus on biological outcomes in our experiments. Our biomaterials are diverse in nature: we use synthetic polymers like poly(ethylene glycol), natural extracellular matrix molecules like collagen and hyaluronic acid (the same ingredients you might find in fancy lotions and face creams), and designer peptides that we make from the same building blocks as the natural extracellular matrix, but which allow us customized control of chemical and physical parameters.
We use these materials for several purposes, but one unifying feature is that they mimic the mechanical and physical behavior of real brain tissue. Most folks haven’t seen or touched a brain – but living brain tissue is just barely a solid. (There’s a reason why it’s almost completely surrounded by a rigid, dense skull.) So we make materials called hydrogels, which like the brain, are soft and squishy and > 90% water. Hydrogels provide an environment for cells to grow, nutrients and waste to diffuse to and from the cells, and with the proper engineering, serve as a cue to direct stem cell fate down desired lineages.
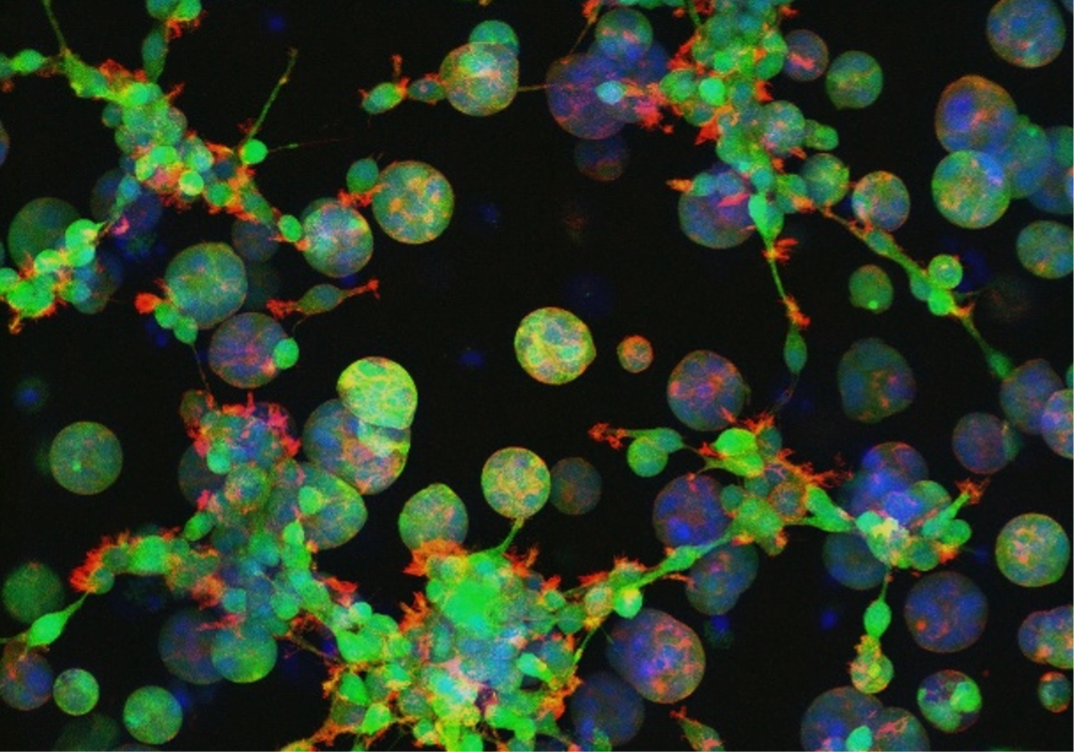
Culturing neural stem cells on stiff surfaces such as “traditional” tissue culture plastic, which is more stiff than bone, biases the cells to do unnatural things (1). Culturing those cells within a soft “goo” helps them retain a healthy, normal status. With proper “goo engineering,” our lab creates hydrogels that can selectively guide the differentiation of stem and progenitor cells into the aforementioned oligodendrocytes that are critical to myelination (2). We can then use these bits of engineered tissue to study how oligodendrocytes and myelin respond to mechanical damage like a traumatic brain injury in collaboration with experts at Virginia Tech (3).
Alternatively, we can make a hydrogel flow like a liquid during syringe injection, just like squeezing a ketchup bottle. Giving the hydrogel molecules the ability to dance and flow, protects cells from mechanical damage (4) the way an armored storm-chasing vehicle might protect occupants from various external forces when experiencing a tornado. After injection, the biomaterial rapidly turns back into a hydrogel, providing stem cells with just the right microenvironment to kickstart regeneration and rebuild lost brain tissue – at least that’s what we are working toward.
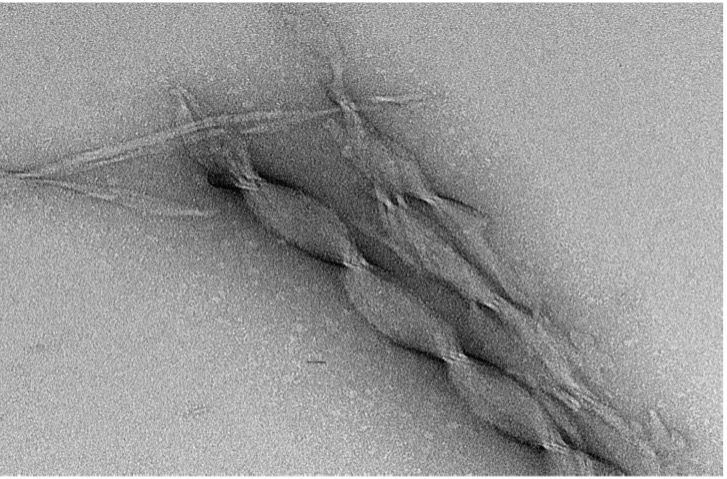
We pay for most of our research through grants from federal agencies such as the National Science Foundation or National Institutes of Health, with student researcher stipends typically the biggest part of the budget proposal by far. Though many applications are unsuccessful – only about 10% of those federal grant proposals get funded – discovering new things WITH students keeps me coming back for more. They bring truly ingenious and fearless elements to our research team. While writing research proposals for funding is often what keeps me up nights, mentoring these folks as they make impactful neural engineering discoveries is most certainly what gets me up in the mornings.
Click to watch a UVA video on the Lampe Research Group: https://www.youtube.com/watch?v=TViUvt0rIg0
(1) KJ Lampe*, RG Mooney*, KB Bjugstad, MJ Mahoney, “Effect of macromer weight percent on neural cell growth in 2D and 3D nondegradable PEG hydrogel culture,” Journal of Biomedical Materials Research Part A: 94A, 1162-1171 (2010). (LINK) *contributed equally to this work
(2) E Meco, WS Zheng, A Sharma, KJ Lampe, "Guiding Oligodendrocyte Precursor Cell Maturation with Urokinase Plasminogen Activator-degradable Elastin-like Protein Hydrogels," Biomacromolecules. 21 (12), 4724-4736 (2020). LINK
(3) RA Mazur, R Yokosawa, PJ VandeVord, KJ Lampe, "The Need for Tissue-engineered Models to Facilitate the Study of Oligodendrocyte Progenitor Cells in Traumatic Brain Injury and Repair," Current Opinion in Biomedical Engineering. 22: 100378 (2022). LINK
(4) JD Tang, Roloson EB, Amelung CD, KJ Lampe, "Rapidly Assembling Pentapeptides for Injectable Delivery (RAPID) Hydrogels as Cytoprotective Cell Carriers," ACS Biomater. Sci. Eng. 5: 2117-2121 (2019) . LINK
- Testing, Diagnosing, and Treating Primary Aldosteronism: A Leading Cause of Hard-to-Treat Hypertension
- Life at the Top: Climate Change in Utqiaġvik, Alaska
- The Only Thing We Have to Fear is Fear Itself
- UVA Club of Tidewater: Hoos at Harbor Park
- UVA Club of Washington, DC: Diplomacy & Dialogue with Ambassador Mull
- UVA Club of Middle Tennessee: An Evening with Julie Myers (Col ’90)